Radioactivity
Henry Becquerel made the discovery of natural radioactivity in 1896. He discovered that exposure to double potassium and uranium sulfates causes photographic plates to become black. Becquerel came to the conclusion that uranium emits a unique type of ray that is known as Becquerel rays after making additional observations in this regard. Pitchblende emits radiation that is four times as powerful as uranium, according to Pierre and Marie Curie. The source of the greater radiation was then actively sought after as a result. They were finally successful in finding two new compounds in 1898, which they termed Polonium (84Po210) and Radium (88Ra226).
Natural radioactivity is the spontaneous conversion of one type of element into the other type accompanied by the emission of one or more particles or electromagnetic radiation in the form of alpha particles, beta particles, or gamma radiation.
The substances capable of emitting radiation are called radioactive substances. As a rule, radioactivity is exhibited by the heavy nuclei occurring at the end of the periodic table beyond the lead element. The radiation so emitted by the radioactive elements is termed nuclear radiation.
Nuclear Radiation:
Ernest Rutherford focused on gauging the radiation output of a radioactive substance two years after the discovery of radioactivity in 1828. The setup used for the experiment was as follows:
A set distance between the radioactive material and the detector was used. Between the material exhibiting radioactivity and the detector were placed foils of varying thicknesses, beginning with paper foils that were incredibly thin and progressing to aluminum foils. The relationship between foil thickness and radiation intensity was presented as a graph. The resulting graph is depicted in [Figure 8.30]. Three unique points can be seen on the resulting curve.
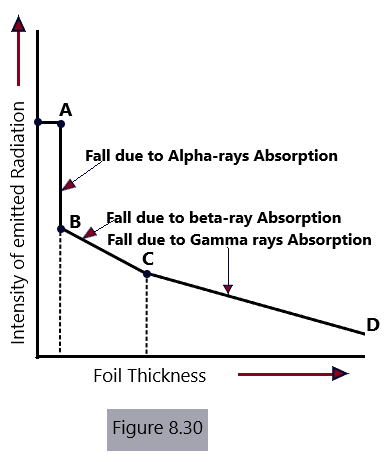
One of the radiation’s less invasive components is quickly absorbed in the region AB of the curve. This element was previously known as alpha Rays.
Along BC, the curve does not abruptly flatten out with distance once the alpha rays have been absorbed by a specific thickness of the foil. Therefore, it signifies the absorption of a piercing radiation component known as beta rays.
Along CD, a change of a similar nature occurs, demonstrating the absorption of the third radiation component. In this area of the graph, the decline of the curve with distance is very gradual, which is consistent with a very penetrating component of the radiation. Gamma Rays are the name given to this element.
In 1899, Rutherford studied the effects of electric and magnetic fields on compounds exhibiting radioactivity, in order to examine the radiation emitted by them. In a deep hollow block of lead, a little amount of a radioactive material is put that exhibits radioactivity. The radiation that hits the cavity’s walls is absorbed, and the leftover energy is transformed into a narrow, parallel beam. The radioactive material is housed in a lead cavity that has been emptied. By positioning two charged parallel plates on either side of the radiation beam, as shown in [Figure 8.31], a strong electric field is applied to the beam of radiation.
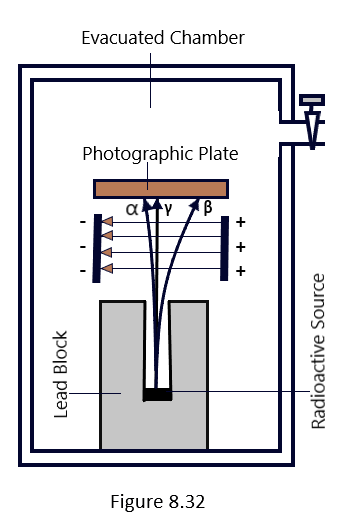
It is discovered that a small portion of the beam slightly bends in the direction of a negative plate, indicating that the plate is made up of dense, positively charged particles known as alpha particles. A significant portion of the beam’s opposite end bends in the direction of the positive plate, indicating the presence of beta-particles, which are light and negatively charged particles. The remaining portion of the beam, however, continues straight and is unaffected by the electric field. Gamma Rays were given their name once it was discovered that they were electromagnetic in nature.
By applying a magnetic field perpendicular to the three different types of nuclear radiation that a substance emits owing to radioactivity, as seen in [Figure 8.33], the three different types of nuclear radiation can also be identified. It demonstrates how the radiation’s course is deflected when a strong magnetic field is present, both inward and perpendicular to the plane of the paper. According to Fleming’s left-hand rule, positively charged particles make up the component that is deflected to the left, while negatively charged particles make up the component that is reflected to the right. These elements were identified as beta and α-rays, respectively. However, the gamma ray, the third component, is unaffected, demonstrating that it is chargeless.
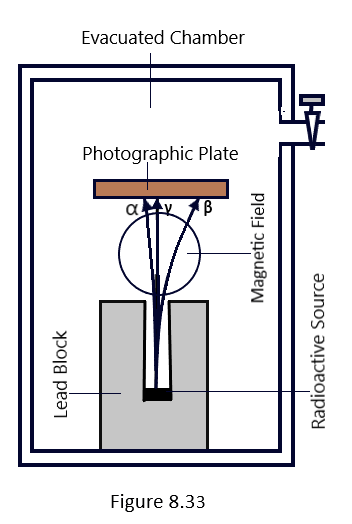
Properties of alpha-rays:
An alpha particle is represented by a helium-4 nucleus consisting of two protons and two neutrons. They are frequently released when certain radioactive isotopes undergo alpha decay. Alpha particles have the following salient characteristics:
Composition: The two protons and two neutrons that make up an alpha particle give it a total charge of +2 (2 protons) and a mass number of 4 (2 protons + 2 neutrons). It is depicted symbolically α.
Energy: Depending upon the substance exhibiting the phenomenon of radioactivity, the velocity of α-particles emitted, lies in the range of 1.4 x 107 m s-1 to 2.05 x 107 m s-1. In other words, the energy of the α- particle emitted during the course of radioactivity of a substance is from 4.19 to 6.78 M eV.
Penetration: Alpha particles are highly energetic particles, even then, they have low penetration capabilities. They can be stopped by a sheet of paper or even the outer layer of dead skin cells. They can travel only a few cm in the air, making them the least penetrating type of ionizing radiation. This is due to the fact that the alpha particles are heavy and massive particles and hence are easily stopped by an aluminum sheet of thickness 0.02 mm or by an ordinary sheet of paper.
Ionization power: The alpha particles because of their relatively large mass and double positive charge, have usually high ionization power. As they move through matter, they produce thousands of ions before being absorbed.
Range in Matter: Due to their relatively large size and high ionization potential, alpha particles due to radioactivity have a short range in matter. They travel only a short distance before losing their energy and coming to a stop. At normal pressure in the air, the range of alpha particles ranges from 3.0 cm to 8.0 cm depending on their initial kinetic energy.
Biological Impact: Due to their low penetration, alpha particles pose minimal external radiation hazards to humans. However, they can be dangerous if materials, emitting alpha particles by radioactivity are inhaled, ingested, or enter the body through open wounds, as they can cause significant damage to internal tissues.
Fluorescence: Alpha particles emitted due to radioactivity, produce fluorescence in the substances like zinc sulphide and barium platinocyanide, when allowed to pass through them and these particles affect the photographic plate.
Scattering: In addition to the properties discussed above, the alpha particles are deflected when subjected to the electric and magnetic fields due to their positive charge. They can also be scattered when they are made to pass through the metal foils.
Induction of Radioactivity: Alpha particles can induce radioactivity in certain elements, called as artificial radioactivity, and can produce nuclear reactions.
Properties of beta-rays:
Beta rays are often called beta particles. They are a type of ionizing radiation emitted during beta decay during radioactivity in certain radioactive isotopes. Beta particles are of two types: (β–) and (β+), as discussed below
Beta-minus (β–) particles:
- A β–-particle is essentially an electron.
- During beta-minus decay, a neutron within the nucleus is converted into a proton, and an electron and an antineutrino are emitted.
- The electron is then ejected from the nucleus with a significant fraction of the speed of light.
- The emitted electron carries a negative charge (−1 elementary charge) and has a relatively small mass compared to a proton or neutron.
- Since an electron is involved in beta-minus decay, the atomic number of the nucleus increases by one while the mass number remains unchanged.
- The ejected electron interacts with matter, losing energy gradually and ionizing atoms along its path. Eventually, it comes to rest after losing all its energy.
Beta-plus (β+) particles:
- A beta-plus particle is a positron or called as positron.
- During β+ decay, a proton within the nucleus is converted into a neutron, and a positron and a neutrino are emitted.
- The positron is the antiparticle of an electron, carrying a positive charge (+1 elementary charge) and having the same mass as that of an electron but with an opposite charge.
- When a positron encounters an electron, they annihilate each other, releasing two gamma-ray photons.
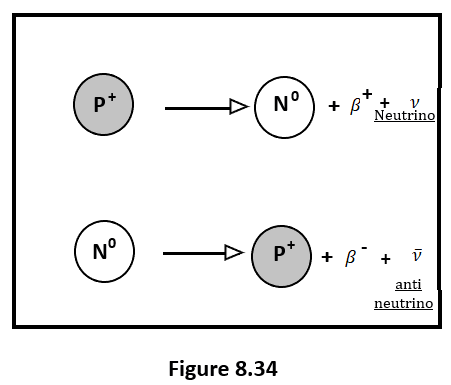
Properties of beta particles:
Charge: Beta particles have been have been observed to be the stream of electrons and carry an electric charge due to their composition as electrons (β-) or positrons (β+) given to be -1.6 x 10-19 C or +1.6 x 10-19 C The charge determines how they interact with electromagnetic fields and matter.
Penetration: Beta particles have moderate penetration capabilities. They can travel through air and some materials, but their range is limited compared to more penetrating forms of radiation like gamma rays.
Mass: Beta particles have a relatively small mass compared to alpha particles (helium nuclei) but are more massive than gamma rays (photons).
Ionizing Ability: Beta particles are ionizing radiation, meaning they have enough energy to knock electrons off atoms as they pass through matter. This ionization can cause chemical changes and damage biological tissues.
Shielding: Beta particles can be effectively shielded by materials such as plastic, glass, or even a few millimeters of aluminum. However, they require thicker and denser shielding (e.g., several centimeters of lead or several meters of concrete) to completely stop them.
Detection: Beta particles can be detected using instruments like Geiger-Muller counters or scintillation detectors. The detectors register the ionization or light produced by the interactions of beta particles with matter.
Energy of β-particles: The velocity of β-particles has been found to range 33 % to 99.8 % of the velocity of light. Although the velocities of these particles are found to be enormous, their average energy is only 2 to 3 M eV. It is due to the reason that the β-particles are the lighter particles with an extremely small mass.
Properties of Gamma rays:
Electromagnetic radiation:
Gamma rays belong to the electromagnetic spectrum, just like visible light, radio waves, microwaves, and X-rays. However, gamma rays have the highest energy and the shortest wavelengths among all electromagnetic waves. These radiations have the same velocity as the light radiations have.
High energy: Gamma rays have the highest energy among all types of electromagnetic radiation. They have energies ranging from hundreds of keV (kilo-electron volts) to several MeV (mega-electron volts). This high energy allows them to penetrate deeply into the matter. *Radioactivity
*
Gamma radiations are highly penetrating. They can penetrate easily through several centimeters thick iron sheet and lead block. *Radioactivity
*
Gamma radiations have a small ionizing power and can produce fluorescence in a substance like willemite.
Gamma radiations are not deflected by an electric and a magnetic field as they do not carry charge. However, they can affect the photographic plate. *Radioactivity
*
Gamma radiations, exhibit heating effect in the surface, exposed to them and even, they can knock out electrons from the metal surfaces on which they fall. *Radioactivity
*
Applications of gamma rays:
Medical Imaging and Radiotherapy: In order to diagnose and track diseases including cancer, heart issues, and neurological disorders, gamma rays are currently being used in imaging techniques with gamma cameras and PET (Positron Emission Tomography) scans. Gamma rays are used in radiotherapy to cure cancer.
Industrial Radiography: Gamma rays are nowadays frequently used in industries and other manufacturing units to inspect the integrity and quality of materials, welds, and structures. It helps a lot
in detecting defects and ensuring the safety and reliability of various industrial components.
Sterilization: Gamma rays are nowadays frequently used in various fields like medical, pharmaceutical, and food industries for sterilization procedures. They efficiently eliminate bacteria, viruses, and other germs, extending product shelf life and guaranteeing their safety for use or consumption.
Homeland Security: At border crossings, seaports, and airports, gamma-ray detectors are widely employed for security screening in order to look for the presence of radioactive materials that could be linked to criminal activity or nuclear threats.
Archaeological and Geological Dating: In techniques like gamma-ray spectroscopy, gamma rays are successively used to estimate the age of rocks and artifacts in geology and archaeology.
Gamma rays are highly ionizing and can be dangerous to living things, thus their usage in a variety of applications necessitates cautious handling and the adoption of safety precautions to safeguard both human health and the environment.
Key points:
- GM are the best for the detection α- particle and β-particles but they are less efficient as for as the detection of gamma rays are concerned. It is because of the reason that the gamma rays are highly penetrating and low ionizing power.
- α- particles are Helium nuclei 2He4 , each having 2-neutrons and 2-protons.
- β-particles are the electrons of nuclear origin released during the process of radioactivity.
- β-particles have a higher value of charge to mass ratio (e/m) than the α- particles have.
- Gamma rays are high energy photons of electromagnetic waves of extremely small wavelengths.
- The β-particles of both types (β–) and (β+) are obtained during radioactivity through nuclear reactions, with the conversion of neutron into protons and vice versa. But the total number of neutrons and protons remain constant at each instant of time.